Background
A remarkable breakthrough in the field of topological quantum matter is the discovery of topological insulators (TIs) with nontrivial bulk band topology and metallic boundary states. The recently discovered MnBi2Te4 combines intrinsic magnetism and nontrivial topology in one material, providing an ideal platform for exploring the topological phenomenon in extreme physical conditions. In the 2D limit, it exhibits the QAH insulator and axion insulator phases for odd-number and even-number of SLs.When FM order is induced by magnetic fields, the parity-time (PT) symmetry is broken and the bulk of MnBi2Te4 becomes a Weyl semimetal. In thin MnBi2Te4 flakes, the bulk Weyl semimetal band develops 2D quantum well states due to the quantum confinement along the c-axis. The topologically nontrivial quantum well subbands lead to a robust Chern insulator state with a quantized Hall plateau that persists to relatively high temperatures for magnetic field μ0H> 8 T.A unique feature of the Chern insulator phase in MnBi2Te4 lies in the negative sign of the Chern number (C = −1) in positive magnetization, which is distinguishable from the C=+1 Chern insulator phase in Cr-doped or V-doped Tis.
What we discover?
In this work, we report transport studies on exfoliated MnBi2Te4 in pulsed magnetic fields up to 61.5 T. Unexpectedly, the C = −1 phase evolves into a zero Hall plateau state characterized by a broad Ryx= 0 plateau and insulating Rxx in an ultrahigh magnetic field. Nonlocal transport measurements and theoretical calculations demonstrate the transport of this zero Hall plateau state is composed of two counter-propagating edge states with opposite Chern numbers, which arise from the FM order and the joint roles of Landau levels and Zeeman effect. The robust zero Hall plateau state represents a topological phenomenon that is unavailable in 2D electron-gas or hole-gas with conventional QH effect.
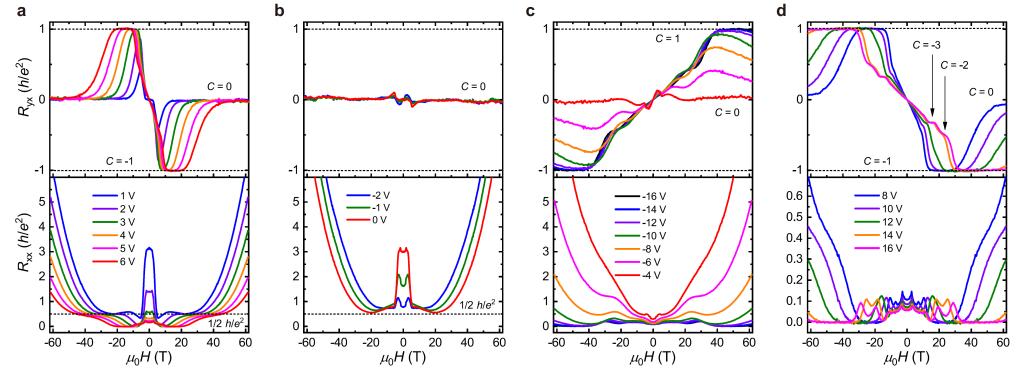
Fig 1:Magneto-transport properties of a 7-SL MnBi2Te4 device in pulsed field up to 61.5T, under various gate voltage Vg. (a)EF close to the center of the gap;(b)EF close to the top of the Valence band;(c) 2D hole gas regime; (d) 2D electron gas regime.
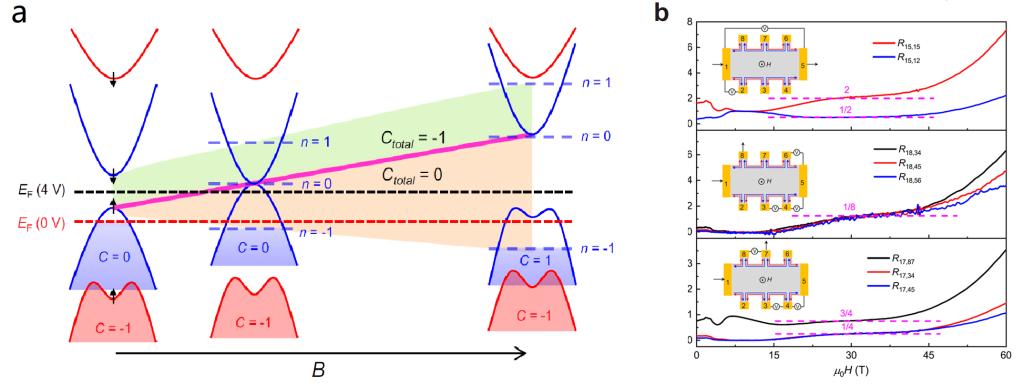
Fig 2:(a) Schematic illustration of the edge state formation and the band structure evolution in a magnetic field with Zeeman-effect-induced band inversion of MnBi2Te4. (b) Two-terminal ,three-terminal, and nolocal measurements in various configurations.
Why is this important?
We realize a zero Hall plateau state in the MnBi2Te4 Chern insulator state when a strong magnetic field is applied. The robust zero Hall plateau against magnetic field and Vg, as well as the nonlocal transport measurements, suggest this state is composed of two counter-propagating edge states that arise from the emergence of a new Chern bandgap. The zero Hall plateau state discovered in the magnetic field in MnBi2Te4 represents a unique quantum transport phenomenon generated by the intricate interplay among intrinsic magnetism, external magnetic field, and nontrivial band topology.
Why did we need WHMFC?
Previous efforts on the Chern insulator mainly focused on its realization in zero magnetic field. An intriguing question that has yet to be addressed experimentally is the fate of the Chern insulator in ultra-high magnetic fields. It is likely that the QAH plateau will not survive forever because extreme conditions may induce other topological phases, as exemplified by the fractional QH effect. Recently, it was demonstrated that helical phases analogous to the QSH phase exist in charge-neutral graphene and non-symmorphic KHgSb crystal in strong magnetic fields, which are characterized by quantized longitudinal resistance (Rxx) and zero Hall resistance (Ryx) plateau in certain magnetic fields and gate voltage (Vg) ranges. Inspired by these discoveries, it is imperative to find out the fate of the Chern insulator phase in MnBi2Te4 in strong magnetic fields, as has been discussed theoretically for magnetic TIs.
Who did the research?
Chang Liu1,2, Yongchao Wang3, Ming Yang4, Jiahao Mao1, Hao Li5,6, Yaoxin Li1, Jiaheng Li1, Haipeng Zhu4, Junfeng Wang4, Liang Li4, Yang Wu6,7, Yong Xu1,8,9, Jinsong Zhang1,9, & Yayu Wang1,9
These authors contributed equally: Chang Liu, Yongchao Wang, Ming Yang.
email: yongxu@tsinghua.edu.cn; jinsongzhang@tsinghua.edu.cn; yayuwang@tsinghua.edu.cn.
1 State Key Laboratory of Low Dimensional Quantum Physics, Department of Physics, Tsinghua University, Beijing, China.
2 Beijing Academy of Quantum Information Sciences, Beijing, China.
3 Beijing Innovation Center for Future Chips, Tsinghua University, Beijing, China.
4 Wuhan National High Magnetic Field Center and School of Physics, Huazhong University of Science and Technology, Wuhan, China.
5 School of Materials Science and Engineering, Tsinghua University, Beijing, China.
6 Tsinghua-Foxconn Nanotechnology Research Center, Department of Physics, Tsinghua University, Beijing, China.
7 Department of Mechanical Engineering, Tsinghua University, Beijing, China.
8 RIKEN Center for Emergent Matter Science, Wako, Saitama, Japan.
9 Frontier Science Center for Quantum Information, Beijing, China.
Acknowledgments
This work is supported by the Basic Science Center Project of NSFC (grant No. 51788104) and the National Key R&D Program of China (grants No. 2018YFA0307100 and No. 2018YFA0305603). This work is additionally supported by the NFSC (grants No. 12004122, No. 51991340 and No. 21975140). This work is supported in part by the Beijing Academy of Quantum Information Sciences (BAQIS) and the Beijing Advanced Innovation Center for Future Chip (ICFC).
Link
https://www.nature.com/articles/s41467-021-25002-x