Background
Shortly after the discovery of high-temperature superconductivity in cuprates, it was found that their normal states exhibit non-Fermi-liquid transport behaviours, such as a linear-in-temperature (T-linear) resistivity and a linear-in-field (H-linear) magnetoresistance (MR). This unusual normal state, called a strange metal, has been observed in many unconventional superconductors including cuprates and Bechgaard salts. In the phase diagram, the strange-metal state often appears in the neighbourhood of the unconventional superconducting dome, indicating that studying the strange-metal state may provide clues towards understanding the microscopic mechanism of unconventional superconductivity. Nevertheless, despite extensive experimental and theoretical efforts over the past decades, the origin of the strange-metal state and its connection with superconductivity remain major puzzles in condensed-matter physics.
Early on, the strange-metal state was considered to be associated with quantum critical physics; yet, in many material families, it was found that T-linear resistivity at low temperatures remains robust over a wide doping range. Such an extended strange-metal regime was further identified by the recent observations of H-linear MR at different doping levels in unconventional superconductors such as BaFe2(As1–xPx)2=, La2–xCexCuO4(LCCO), Tl2Ba2CuO6+δ and (Pb/La)-doped Bi2Sr2CuO6+δ. Intriguingly, a specific H/T scaling of MR was identified in these systems, implying a scale-invariant region where temperature and magnetic field play a similar role in setting an energy scale in transport.
What we discover?
In this work, we investigate this phenomenon in iron-based superconductors—another major class of high-Tc superconductors—in which apart from some fragmented evidence for the strange-metal behaviour in iron pnictides, a comprehensive study of the interplay between strange-metal state and superconductivity has been lacking. By studying the zero- and high-field electrical transport properties of the iron chalcogenide superconductor FeSe, we obtain clear evidence for strange metallicity in this system, including T-linear resistivity, H-linear MR and linear scaling of MR between T and H. An advantage of FeSe is that its superconductivity can be substantially boosted by electron doping, such as surface K dosing, alkali metal/molecule interlayer intercalation and ionic-liquid gating, providing a unique opportunity to explore the evolution of strange metallicity and superconductivity across the phase diagram.
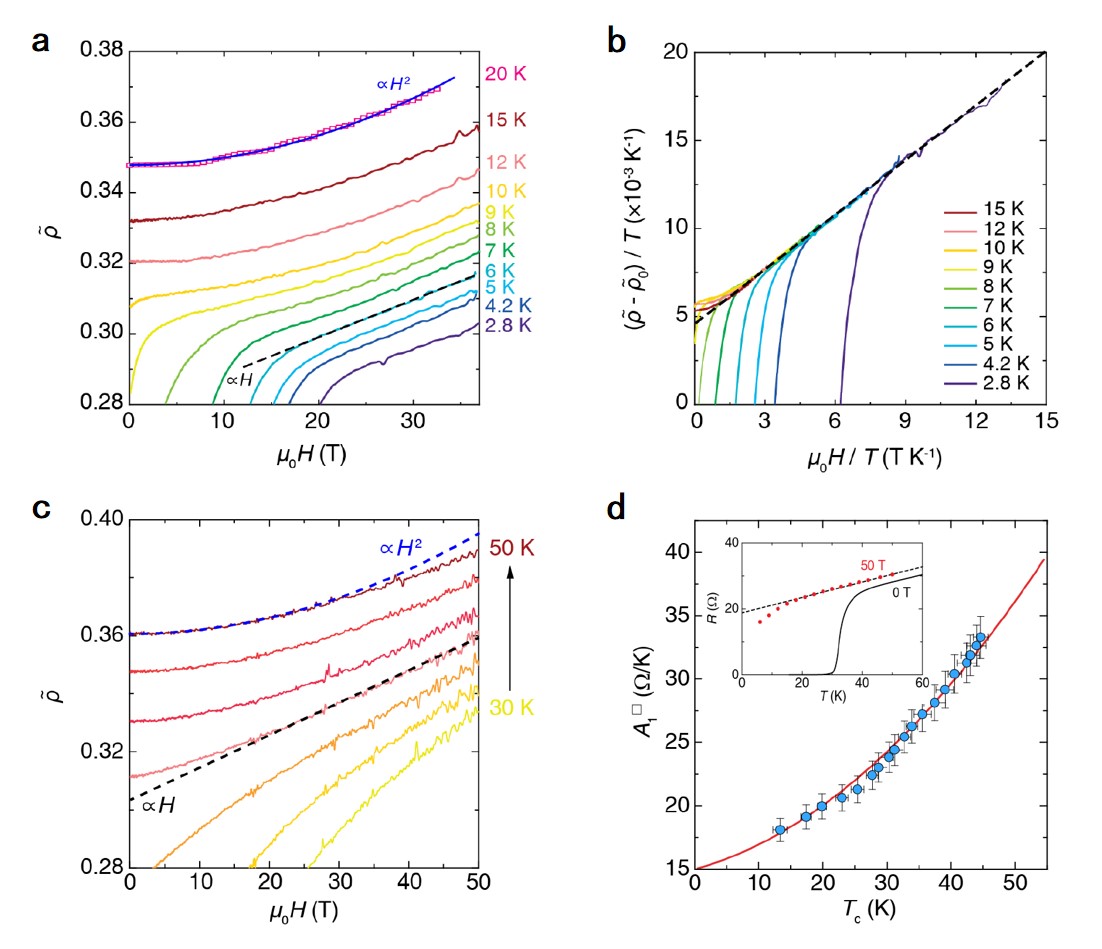
Why is this important?
Here we report the observation of a typical strange-metal behaviour in FeSe, namely, T-linear resistivity, linear-in-field magnetoresistance and universal scaling of magnetoresistance. More importantly, when we tune the superconductivity by ionic-liquid gating, the superconducting transition temperature increases from approximately 10 to 45 K, and the T-linear resistivity coefficient exhibits a quadratic dependence on the critical temperature. This is a ubiquitous feature that describes the relation between these parameters in various systems including overdoped cuprates and Bechgaard salts. This suggests that there may be a universal mechanism underlying the T-linear resistivity and unconventional superconductivity.
Why did we need WHMFC?
Research into high-temperature superconductivity is difficult owing to the complexity and sensitivity of the properties of these materials. Superconducting phase diagrams contain multiple variables, making it difficult to separate and control a single tuning parameter with fine steps to elucidate the entire electronic phase diagram. Moreover, obtaining quantitative expressions that describe the relationship between Tc and other parameters is crucial to understand the fundamental physics, but remains challenging. We tackled this challenge by employing two approaches: first, composition-spread (combinatorial) film fabrication, and second, ionic-liquid gating integrated with in situ electrical and magnetic characterizations. The former led to the discovery of a quantitative relationship between A1 and Tc in an electron-doped cuprate, which lays the foundation for this work. Unveiling additional quantitative relationships with advanced techniques could bring us closer to the truth of high-temperature superconductors.
Who did the research?
1.Beijing National Laboratory for Condensed Matter Physics, Institute of Physics, Chinese Academy of Sciences, Beijing, China
Xingyu Jiang, Mingyang Qin, Xinjian Wei, Li Xu, Ruozhou Zhang, Zhanyi Zhao, Zhongxu Wei, Zefeng Lin, Zhongpei Feng, Fucong Chen, Peiyu Xiong, Jie Yuan, Beiyi Zhu, Yangmu Li, Tao Xiang, Jiangping Hu, Kun Jiang, Qihong Chen, Kui Jin & Zhongxian Zhao
2.School of Physical Sciences, University of Chinese Academy of Sciences, Beijing, China
Xingyu Jiang, Mingyang Qin, Li Xu, Ruozhou Zhang, Zhanyi Zhao, Zhongxu Wei, Zefeng Lin, Fucong Chen, Jie Yuan, Yangmu Li, Tao Xiang, Jiangping Hu, Kun Jiang, Qihong Chen, Kui Jin & Zhongxian Zhao
3.Wuhan National High Magnetic Field Center and School of Physics, Huazhong University of Science and Technology, Wuhan, China
Jiezun Ke, Haipeng Zhu, Ming Yang & Junfeng Wang
4.Anhui Province Key Laboratory of Condensed Matter Physics at Extreme Conditions, High Magnetic Field Laboratory of the Chinese Academy of Sciences, Hefei, China
Qimei Liang, Chuanying Xi & Zhaosheng Wang
5.Songshan Lake Materials Laboratory, Dongguan, China
Zhongpei Feng, Jie Yuan, Qihong Chen, Kui Jin & Zhongxian Zhao
6.Beijing Academy of Quantum Information Sciences, Beijing, China
Tao Xiang
Acknowledgments
We thank R. Yu, H. Xie, D. Zhang, G. Xu, Z. Zhang, G. Zhang, K. Liu, X. Wu, G. He, L. Liang and B. Leridon for stimulating discussions. This work was supported by the National Key Basic Research Program of China (2022YFA1403900 (J.H., Q.C., K. Jiang), 2022YFA1403000 (Q.C.), 2021YFA0718700 (K. Jin), 2017YFA0302900 (T.X., K. Jin)), the National Natural Science Foundation of China (12225412 (K. Jin), 11834016 (K. Jin, J.Y.), 11888101 (T.X.), U1832214 (M.Y., J.W.), 11874359 (Z.W.), 11927808 (K. Jin, J.Y.), 11961141008 (B.Z.) and 12274439 (Y.L.)), the Beijing Nova Program of Science and Technology (Grant No. 20220484014 (Q.C.)), the Strategic Priority Research Program (B) of the Chinese Academy of Sciences (XDB25000000 (K. Jin), XDB33000000 (Q.C.)), Beijing Natural Science Foundation (Z190008 (K. Jin, J.Y., Q.C.)), CAS Project for Young Scientists in Basic Research (2022YSBR-048 (K. Jin, J.Y., Y.L.)). The Key Area Research and Development Program of Guangdong Province (grant no. 2020B0101340002 (K. Jin)). A portion of this work was performed on the Steady High Magnetic Field Facilities, High Magnetic Field Laboratory, Chinese Academy of Sciences, and supported by the High Magnetic Field Laboratory of Anhui Province. The pulsed field measurements were performed at Wuhan National High Magnetic Field Center, Huazhong University of Science and Technology.
Link
https://www.nature.com/articles/s41567-022-01894-4